The failure of lead-acid batteries can be attributed to various factors, including vulcanization, water loss, thermal runaway, shedding of active substances, plate softening, etc. In the following sections, we will introduce and analyze each of these factors individually.
1. Vulcanization
The charging and discharging process of lead-acid batteries involves an electrochemical reaction. Lead sulfate is generated during discharge, and during charging, lead sulfate is reduced to lead oxide.
While this electrochemical process is normally reversible, lead sulfate has a tendency to crystallize. When the concentration of lead sulfate in the battery’s electrolytic solution is too high or the static idle time is prolonged, it forms clusters and small crystals.

These small crystals attract surrounding lead sulfate, forming large inert crystals, disrupting the original reversible cycle and leading to irreversible lead sulfate. solution is too high or the static idle time is prolonged, it forms clusters and small crystals. These small crystals attract surrounding solution is to charging crystallized lead sulfate results in its failure to be reduced to lead oxide; instead, it is adsorbed on the grid plate.
This leads to a reduction in the grid plate’s working area, heat loss in the lead-acid battery, and a decrease in battery capacity, a phenomenon known as sulfurization. Vulcanization can also cause short circuits, relaxation and shedding of active substances, grid plate deformation, and fracture, among other complications.
Vulcanization is an inevitable process as long as lead-acid batteries are in use. However, lead-acid batteries in certain applications have a longer lifespan compared to those used in electric bicycles due to a less conducive working environment for vulcanization.
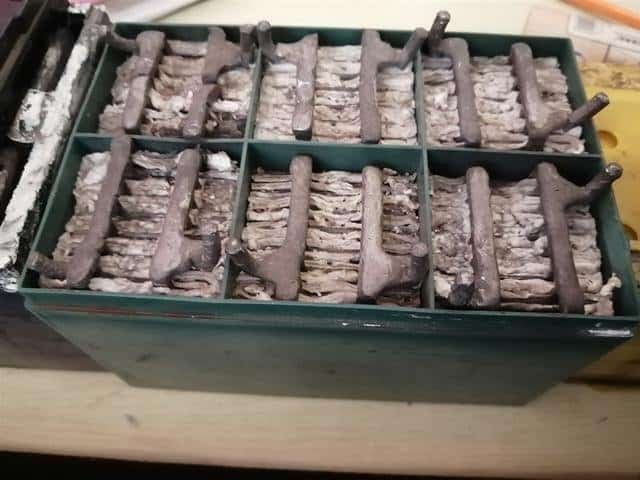
In contrast to automotive starting batteries, which are continuously in a floating charge state after ignition and discharge, electric vehicle batteries accumulate a significant amount of lead sulfate during discharging. If an electric vehicle is deeply discharged, the concentration of lead sulfate is high, and charging the battery promptly after use becomes challenging. Failure to charge the discharged lead sulfate in a timely manner results in crystal formation.
The cycle life of lead-acid batteries varies significantly based on discharge depth. Deeper discharge depths result in fewer cycles, while shallower discharge depths lead to more cycles. Test results indicate the following relationship between discharge depth and cycles:
During continuous high current cycles of 70% 1C charging and 60% 2C discharging in some lead-acid batteries, conditions for generating large lead sulfate crystals are destroyed, mitigating the damage caused by lead acid battery vulcanization.
However, if the test is interrupted prematurely, issues related to lead-acid battery vulcanization may surface. Due to the battery’s substantial weight, some users frequently charge the battery after several use and discharge cycles. Failure to promptly charge the battery after discharge can lead to severe sulfurization of the lead-acid battery.
Additionally, the relatively high proportion of sulfuric acid in lead-acid batteries is a crucial factor in their sulfurization. Sulfurization impairs the negative plate’s ability to circulate oxygen, accelerating water loss. Consequently, a higher sulfuric acid proportion in lead-acid batteries increases the likelihood of lead-acid battery vulcanization. While the degree of sulfurization may vary, its impact on the lifespan of lead-acid batteries is universal.
2.Water Loss
One fundamental principle of sealed lead-acid batteries is that, following oxygen evolution from the positive plate, hydrogen evolves directly to the negative plate, where it is reduced to water. The parameter used to assess the technical index of lead-acid batteries is termed “sealing reaction efficiency,” and this phenomenon is referred to as the “oxygen cycle.” This process ensures minimal water loss in lead-acid batteries, achieving a “maintenance-free” status where no water addition is required. However, the oxygen cycle of sealed lead-acid batteries is compromised in electric bicycles, resulting in significant water loss from the battery.
To achieve a full charge within 8 hours, during the three-stage constant voltage current limiting charging, the constant voltage of a 36V charger is set at 44.4V. With three single batteries, each having 18 cells (equivalent to 2.466V per cell), the positive plate’s oxygen evolution voltage is 2.35V, and the negative plate’s hydrogen evolution voltage is 2.42V.
To reduce the indicated charging time, some charger manufacturers have increased the current from constant voltage to floating charge. After the charging indicator signals full charge, the battery may not be fully charged, compensating by increasing the floating charge voltage. Consequently, many chargers have a floating charge voltage 2.35V higher than the single grid voltage, leading to continued oxygen evolution during the floating charge stage. However, the poor oxygen cycle of lead-acid batteries results in constant exhaust during the floating charge stage. manufacturers have increased the current from constant voltage to floating charge. After the charging indicator signals full charge, the battery may not be fully charged, compensating by increasing the floating charge voltage. Consequently, many chargers have a floating charge voltage 2.35V higher than manufacturers have increased the current from constant voltage to floating charge. After the charging indicator signals full charge, the battery may not be fully charged, compensating by increasing the floating charge voltage. Consequently, many chargers have a floating charge voltage manufacturers have increased the current from constant voltage to floating charge. After the charging indicator signals full charge, the battery may not be fully charged, compensating by increas manufacturers have increased the current from constant voltage to floating charge. After the charging indic
A 36V lead-acid battery group consists of three single cells, each with six cells, totaling more than 15 positive and negative grid plates per cell. A battery group comprises at least 270 solder joints, and if even one-thousandth of these joints is defective, one out of every four battery groups will be disqualified. Lead-calcium plates, prone to solder joint issues due to calcium precipitation, are often used by battery manufacturers. The low antimony alloy plates used for these batteries have a lower gas evolution voltage, leading to increased air output and more significant water loss.manufacturers. Th
While the standard specific gravity of sulfuric acid for floating lead-acid batteries should be between 1.21 and 1.28, batteries for electric bicycles, designed for large capacity and high current discharge, often have sulfuric acid with a specific gravity of about 1.36 to 1.38. The higher sulfuric acid proportion intensifies battery sulfurization, impacting its oxygen circulation ability and accelerating water loss. This loss of water leaves behind a higher proportion of sulfuric acid, making the lead-acid battery more susceptible to vulcanization.
Thus, sulfurization increases water loss, and increased water loss, in turn, exacerbates sulfurization. For users, ensuring proper sealing is essential to prevent the unimaginable consequences of acid spillage. However, excessively promoting the “maintenance-free” concept in the electric vehicle domain may be inappropriate.
3.Thermal Runaway
After the charge of a lead-acid battery reaches 70%, the polarization voltage increases, initiating a gradual rise in the side reaction of charging and the electrolysis of water. Once the charged cell voltage hits 2.35V, oxygen is produced at the positive plate, and at 2.42V, the negative plate begins producing hydrogen. During this time, the charged electric energy is converted into chemical energy, increasing the electrolytic water conversion. Gas evolution during charging depends on the charging voltage, and the amount of gas evolution is influenced by the charging current after reaching the gas evolution voltage.
Therefore, during the charging process, as the voltage approaches its maximum under constant voltage, and the charging current maintains the current limit value, gas evolution is at its peak. After entering constant voltage, the charging current should gradually decrease, resulting in a reduction in gas evolution. Charging, being an exothermic reaction, is generally designed for lead-acid batteries to control temperature rise.
However, after substantial gassing of a lead-acid battery, the compounded oxygen into water on the negative plate generates more heat than during charging. Sealed lead-acid batteries aim for good oxygen circulation ability at the negative plate, but this circulation generates heat. Thus, the oxygen cycle is a double-edged sword, providing the advantage of reduced water loss but with the disadvantage of heating the battery.
Under constant voltage charging, the oxygen circulating current also contributes to the charging current, slowing the rate of decline of the charging current. Nevertheless, the heating of the lead-acid battery may cause the charging current to decrease more slowly, or even rise reversely. Battery heating can lead to an increase in the charging current once it reverses, resulting in a continuous rise to the current limit. This generates high heat, accumulating until the battery shell is thermally softened and deformed, causing the battery to bulge due to the internal high air pressure.
This is the battery thermal runaway that damages the battery. Once the lead-acid battery is seriously swollen, problems such as acid leakage and air leakage also occur, leading to acute battery failure. There are various factors that induce battery bulging. If the charging voltage is high and gas evolution is substantial, thermal runaway is likely to occur.
If a group of batteries or a single-cell battery lags significantly, and the constant voltage value of charging remains unchanged, other single-cell batteries will experience relatively high charging voltage, leading to the problem of thermal runaway. To reduce the likelihood of thermal runaway, many charger manufacturers decrease the constant voltage to 43 volts, but this inevitably results in undercharging. manufacturers decrease the constant
Another reason for the heat generated during the charging of lead-acid batteries is sulfurization. Sulfurization directly increases the internal resistance of the batteries, subsequently causing increased charging heat. This heating also elevates the oxygen circulating current, making batteries with severe sulfurization more prone to thermal runaway.
Analysis of the failure modes of lead-acid batteries for electric bicycles reveals that 90% of failed batteries exhibit severe water loss. Gel batteries experience less water loss than ordinary batteries, potentially resulting in a longer lifespan. Gel batteries also exhibit less self-discharge during storage, as evidenced by the ratio of capacity decline after storage. Despite having a certain internal pressure, gel batteries experience less water loss due to gas evolution than common batteries.
Each time the valve opens, gas dissipates some heat. Colloid lead-acid batteries have fewer valve openings than ordinary lead-acid batteries, and their advantage lies in reduced water loss. However, fewer gas and water losses, fewer valve openings, and less heat dissipated from the battery result in higher internal temperature rise compared to ordinary batteries. Increased internal temperature, in turn, leads to higher self-discharge and heat generation.
Thus, during summer with higher ambient temperatures, due to the reduced gas evolution level, the gas evolution amount is at its maximum, resulting in a higher temperature rise. This increases the likelihood of colloidal lead-acid batteries entering thermal runaway.
4.Active Substance Falling Off and Electrode Plate Softening
The active substance of the positive plate of a lead-acid battery comprises lead oxide α-PbO2 and β-PbO2. Alpha-PbO2, being hard and small in capacity, attaches to the electrode plate in the form of holes to expand the electrode plate area and provide support. Beta-PbO2, attached to the skeleton composed of PbO2, has a much stronger charging capacity ratio than alpha-PbO2. Lead sulfate forms after lead oxide discharge, and in a strong acid environment, β-PbO2 undergoes active substance shedding while α-PbO2 falls off.
Several factors contribute to the shedding of active substances:
- Uneven distribution of active substances on the electrode plate leads to falling off due to different expansion tension during discharge.
- Overdischarging and undervoltage of the lead-acid battery reduce β-PbO2, causing α-PbO2 to participate in the discharge reaction, generating lead sulfate.
- Expansion tension of sulfide crystals growing on the electrode plate can also lead to the shedding of active substances.
Once the positive plate softens, the porous structure supporting it is destroyed. The compacted porous structure reduces the real area involved in the reaction, decreasing the capacity of the lead-acid battery. Preventing overdischarge and inhibiting sulfurization are crucial measures to control positive plate softening. The life of a normally used lead-acid battery depends on positive plate softening without dehydration, vulcanization, and overdischarge.
5.Short Circuit
Lead-acid battery short circuit refers to the connection of positive and negative pole groups inside the battery. To increase lead-acid battery capacity, the number of plates in electric vehicle batteries is generally increased, resulting in a thinner separator compared to other batteries. The lead sulfate crystals on the negative plate grow, and after charging, some lead sulfate is left in the separator. Once the lead sulfate in the separator is reduced to lead, the lead-acid battery experiences micro short circuit, known as “lead bridging.”
This small short circuit causes a lag in the single grid voltage, and in severe cases, single grid short circuit may occur. The expansion and shedding of active substances on the plate can also cause the connection of positive and negative plates.
6.Imbalance
While many lead-acid batteries perform well in individual tests, serial lead-acid batteries may face challenges due to original grouping errors such as differences in capacity and open circuit voltage. Batteries with higher voltage may lose water during charging, while those with lower voltage may be undercharged. This leads to overdischarge in batteries with low voltage, causing lead-acid battery vulcanization. As the cycle of charging and discharging continues, the sulfurized monomer of the lead-acid battery is more susceptible to sulfurization, expanding this difference and ultimately affecting the life of the entire battery group.
7. Unable to Charge
The terminal discharge voltage of a 12V lead-acid battery is 10.5V. If forced discharge occurs below this terminal voltage, the lead-acid battery has a high chance of losing its recharging capacity. Electric vehicle controllers usually have a protection device. When the lead-acid battery reaches the termination voltage, the protection device disconnects the circuit forcibly. However, if the protection device malfunctions or the battery voltage rises after a power failure, the protection device may not judge correctly.
8. Self-discharge of Lead-acid Battery
The phenomenon where fully charged lead-acid batteries gradually lose power when not in use is called self-discharge. Self-discharge is inevitable, and under normal conditions, the daily discharge rate should not exceed 0.35% to 0.5%. The main reasons for the self-discharge of a lead-acid battery include:
- The electrode plate or electrolyte contains impurities, leading to the generation of a potential difference between the impurities and the electrode plate or among different impurities. This creates a local battery within the circuit formed by the electrolyte, generating a localized current that discharges the lead-acid battery.
- The diaphragm is damaged, resulting in a short circuit between the positive and negative plates.
- Electrolyte or water on the surface of the lead-acid battery shell acts as a conductor between the poles, causing the discharge of the lead-acid battery.
- Excessive shedding of active substances, with subsequent deposition at the bottom of the battery, leads to a short circuit of the electrode plate and discharge.